How Total Harmonic Distortion Affects Your Power System
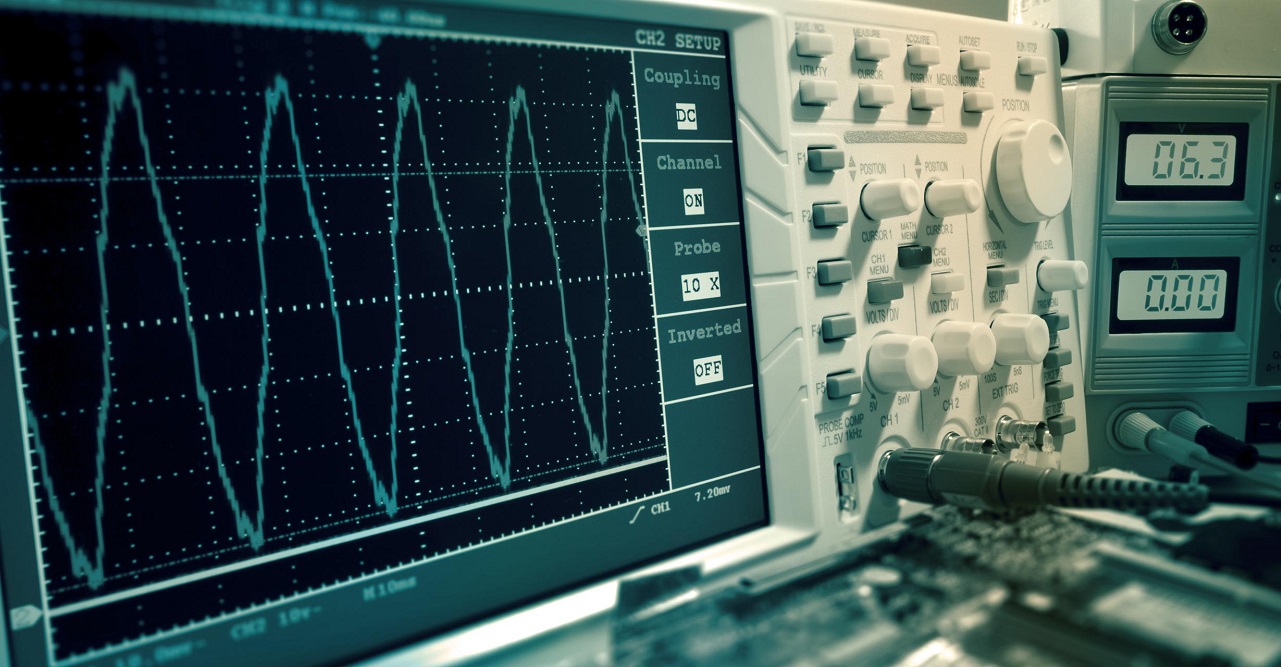
It would be nice if, at least in the US, the power that came from the wall was truly noise free. Unfortunately, this is not the case, and although a power system can appear to output a clean sine wave, zooming into an oscilloscope trace or using an FFT will tell you a different story. When you take dirty power, put it through rectification, and then pass it through a switching regulator, you introduce additional noise into the system that further degrades power quality. If you’re a power supply or power systems designer, then you know the value of supplying your devices with clean, noise-free power.
Multiple disciplines in engineering use total harmonic distortion as a metric for quantifying the quality of power supplied to your electronics. So where does this harmonic content come from, and what can you do about it in your design? The key is to control or compensate for nonlinearity in your circuit design, and following some basic layout practices can help ensure power supply noise is contained.
Diagnosing Harmonics in Power Systems
When a power supply or power converter draws power from AC mains or a generator, it’s common for the waveform to be a bit distorted when viewed on an oscilloscope. The distorted voltage/current that gets measured from mains or generator power deviates from an ideal sine wave in terms of its magnitude, although slight variations in frequency and phase can also occur. In power systems engineering, THD and power factor are most commonly used to quantify the extent to which an AC waveform deviates from an ideal sinusoidal waveform. The latter can be defined in terms of the former and can be measured in the time domain.
Thanks to Fourier analysis, we can write the voltage (or current) of an AC waveform as a sum of some fundamental frequency (50 or 60 Hz in power systems) and all of its harmonics.
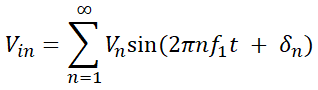
Whenever the harmonics (components with n > 1) are non-zero, you have harmonic distortion. Harmonics in power systems can have very small amplitudes, reaching -30 dB or less than the fundamental frequency, but the power they carry adds up over hundreds of harmonics. So, we need some way to diagnose the total amount of distortion in our waveform.
Total Harmonic Distortion
The AC waveform in power systems will always have some harmonic content that creates distortion, but what’s important is whether this distortion has a noticeable effect on efficiency and signal quality at the system output. Total harmonic distortion is defined as the sum of squared peak voltages contained in the harmonics to the voltage of the fundamental waveform:
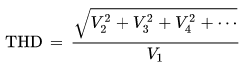
Standards like IEEE 519-2014, IEC 61000-3-6, and NEMA IS07 P1-2019 specify acceptable limits on harmonic content in power systems with THD targets. The above equation is the standard metric used to define THD, although other definitions include noise in the numerator (THD + N) or harmonics in the denominator as a point of reference. The ideal AC waveform will have a THD value of zero (i.e., no harmonics).
One thing that is important to note is that harmonic content in a power system, whether coming from the grid or generated by a regulator, can be measurable up to very high harmonics. In signal integrity, we usually only talk up to the 5th or 7th harmonic, but power systems can have noise spanning dozens of harmonics.
Relation to Power Factor
The main concern regarding harmonics in a power system is power efficiency in the power regulator/converter section, which is a measure of efficiency. This is quantified using the power factor, or the ratio of the voltage (or current) in the fundamental frequency to the total power carried by all frequencies. This can be rewritten in terms of THD and phase angle between the mains voltage and current.
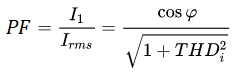
Power factor is a measure of efficiency. When the phase angle above is zero, the power is dissipated at the load as real power, rather than as reactive power. The phase angle will depend on both the load impedance (any reactance) as well as the THD value of the input power. At some point, you will only get the phase difference so small; ideally, you want to get THD as small as possible to prevent low power factor.
Identifying Total Harmonic Distortion
The simplest way to identify harmonic content in your power system is with an oscilloscope that can perform an FFT. As an example, consider the waveform shown below. This is part of a 60 Hz 110 VAC sinusoid. Some glitches can be seen along the crest of the waveform, equating to about 5 V of dropout.
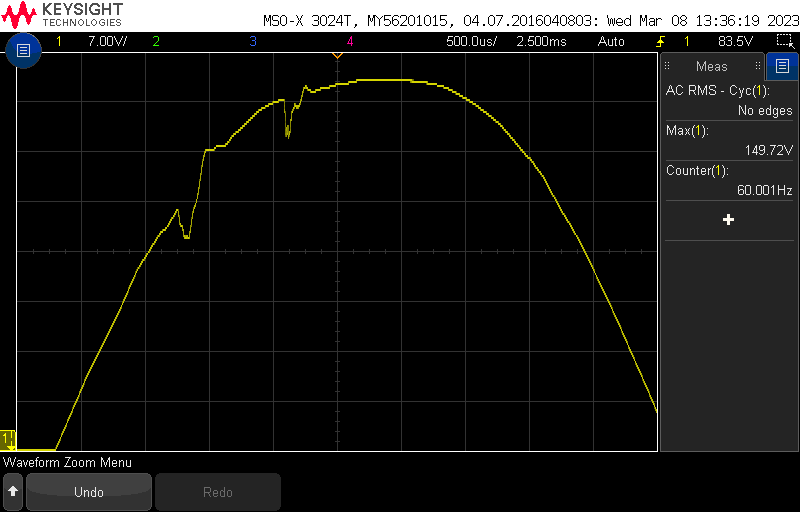
The harmonic content can be seen in an FFT of the above waveform:
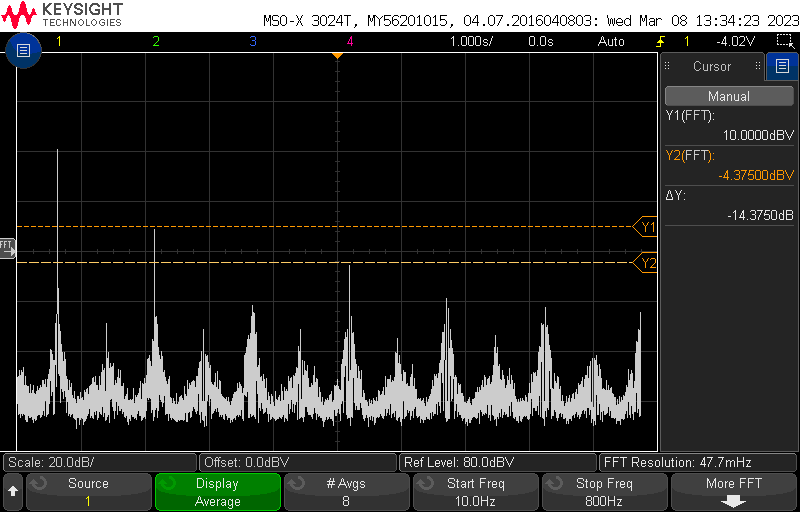
If we can remove this harmonic content, we can clean up this AC wave and ensure higher efficiency power conversion.
Reducing Total Harmonic Distortion in a PCB
There are some steps that can be taken in circuit design and in the PCB layout to remove power system interharmonics that produce harmonic distortion. From the circuit design perspective, you have two options:
- Filtering: The optimal path forward here is to shunt high frequency noise on the input to ground with a large capacitor. Make sure you’ve set the band edge between the first and second harmonic. Some systems may use a pi filter, which along with the output inductor in a switching converter, will form a 4th order filter with high rolloff.
- PFC circuit: This circuit is used in high-current switching converters to compensate for the pulsed current drawn by a switching regulator. It effectively involves recreating the sinusoidal current draw by switching a MOSFET in continuous conduction mode, so the current draw will closely match the voltage supplied by the system.
Filtering Component Placement
Where you place filtering elements in your regulator section will affect the effectiveness of your THD reduction strategy. The first point where EMI filtering should be placed is at the main power input. For AC/DC or DC/DC systems, this will provide low-pass filtering, so it will remove some differential and common-mode harmonic content, thus reducing total harmonic distortion.
Since circuits like PFCs and high power converters use switching elements, these can generate new harmonic content that also needs to be filtered alongside harmonics in the input power. Placement of capacitors near these elements is an important aspect of ensuring filtering with low inductance. Proper placement of capacitors for filtering ensures you do not unintentionally add a pole to the PFC transfer function, which will prevent any gain and oscillation in the circuit at high frequencies.
To do this, you’ll need to place input capacitors with tight loops so that there is low parasitic inductance on the signal path. This will create an effective high-pass filter back to ground. Remember the 3-capacitor guideline used for bypass/decoupling capacitors on high-speed digital ICs? A similar idea applies here to provide a low-pass filter for sourcing power to MOSFETs.
What About Parallel MOSFETs?
Be careful when working in power systems that use MOSFETs in parallel as they have their own placement strategies needed to prevent oscillations during driving. This is normally handled by adding a small amount of resistance to provide damping, rather than trying to filter such an oscillation and any harmonics it generates with filtering. You can read more about placement near parallel MOSFETs in this article.
When you need to design power systems with low total harmonic distortion, low EMI, and safe placement of components, make sure you use the best set of PCB layout features in Altium Designer®. When you need to examine conducted EMI from power systems that contain harmonics or interharmonics, you can use the integrated SPICE package or import your design into a field solver to perform a range of SI/PI/EMI simulations. When you’ve finished your design, and you want to release files to your manufacturer, the Altium 365™ platform makes it easy to collaborate and share your projects.
We have only scratched the surface of what’s possible with Altium Designer on Altium 365. Start your free trial of Altium Designer + Altium 365 today.